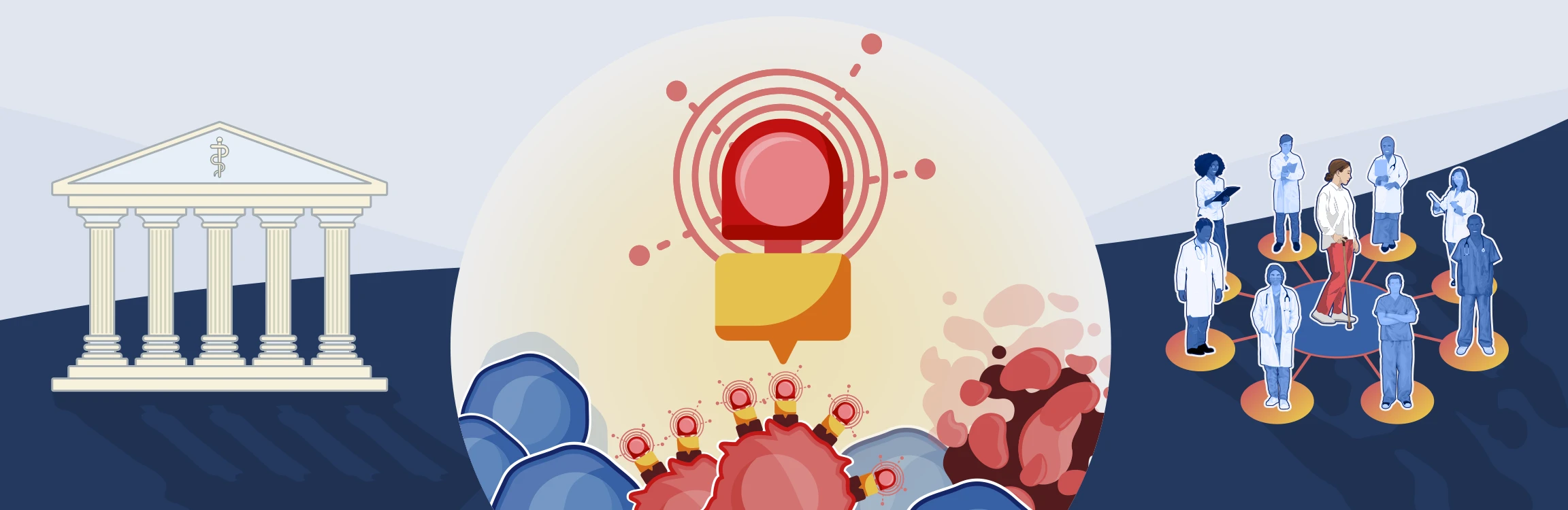
Foundations of theranostics
Radioligand therapy (RLT) and radioligand imaging (RLI) offer a novel, theranostic approach to targeting and treating certain types of cancer.1 This section of the Novartis RLT Institute is designed to help health care professionals (HCPs) understand the basic scientific foundations of RLT. By focusing on the science, we aim to demystify radiation and safety concerns and build confidence in offering patients this approach to precision medicine in cancer.
Radiation science fundamentals
Considerations for radioligand design and selection
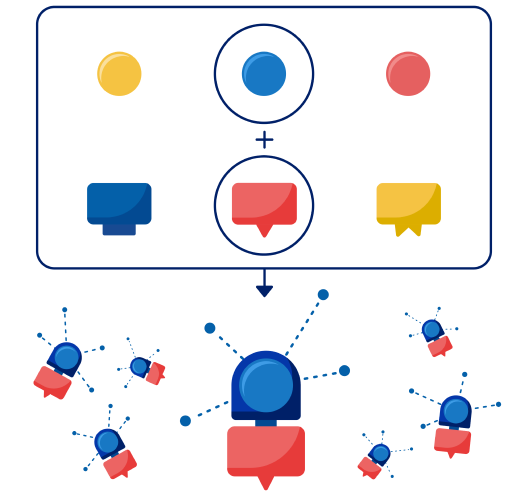
The design of radioligands for imaging and therapy is based in the scientific principles of radiochemistry, physics, and biology. It carefully balances the properties of the radionuclide and the ligand. This requires consideration of target specificity, radioactive isotope properties, pharmacokinetics, and ligand stability. All of these factors enable both therapeutic efficacy and safety.2,3
Radioligands are molecules, usually peptides, proteins, antibodies, or their fragments, that are labeled with a radioactive isotope and specifically target receptors or other biomarkers overexpressed on the tumor cells.4 The type of radiation emitted by the isotope determines the biological effect on the tumor and surrounding tissues.5 The radioligand must have a high binding affinity for its target receptor to enable sufficient localization of the RLT in the tumor, while helping to limit off-target binding to normal tissues.2,4
Radiation biology: The foundation of radioligands
Ligand and target selection
Effective target selection for radioligands requires careful consideration of factors that influence specificity, biodistribution, and therapeutic efficacy.6
Optimal ligands and targets are based on several key criteria:
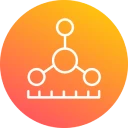
Molecule size: The size of the targeting molecule influences its biodistribution, residence time, and clearance from the body. The residence time of the targeting molecule is strategically paired with the half-life of the isotope to enable effective therapy7,8
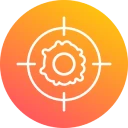
Target specificity: Each radioligand is designed to target a specific biomarker, depending on the tumor type7
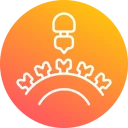
Expression level: High target expression is crucial to enable binding efficiency and therapeutic delivery9
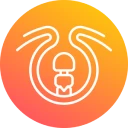
Potential for internalization: Internalization of the radioligand into the cell upon binding to the receptor can influence the retention of radionuclides at the target site. Agonists can promote internalization, while antagonists may not, impacting therapeutic strategy2,3
Biological half-life, biodistribution, and physical half-life
The biological half-life of a radioligand—the time required for its amount in the body to reduce by half through biological elimination—is critical for understanding its biodistribution.7
The physical half-lifes of radionuclides vary widely, which provide a versatile array of theranostic tools.7,10 For example, radionuclides with shorter half-lifes are ideal for diagnostic imaging, as their rapid decay delivers minimal radiation exposure while providing sufficient activity for accurate visualization with positron emission tomography (PET) imaging.11 Radioisotopes used for imaging generally have shorter physical half-lifes and are thus required to be prepared locally at in-house or nearby nuclear pharmacies.12
In contrast, therapeutic radionuclides like 177Lu are selected for their intermediate physical half-life, allowing them to deliver a therapeutic dose that remains active long enough to damage tumor cells effectively while helping to limit exposure and damage to healthy tissues.10 Radioligands with these intermediate half-life radioisotopes can be manufactured centrally and shipped to sites for use.13
Physical half-life
Short
Long

Imaging14:
68Ga (68 minutes)
18F (110 minutes)
64Cu (12.7 hours)
Therapy15-17:
177Lu (6.7 days)
131I (8.0 days)
225Ac (10.0 days)
223Ra (11.4 days)
Residence time
Residence time refers to the average length of time a radioligand remains within a specific organ or tissue in the body. For effective radioligand imaging or therapies, a stable radioligand must persist long enough to achieve sufficient residence time to provide accurate imaging or deliver a therapeutic dose.7,8,10,18
Radiation chemistry considerations
Chemical stability
RLTs need to be stable enough to remain active in the body and consistently deliver the therapeutic dose to the target. This stability enables the radioligand to effectively reach its intended site, stay intact to maintain its function, and help limit effects on healthy tissues.5,8
Radiolabeling process
The radiolabeling process is a critical step in creating RLTs, involving the binding of radionuclides to a targeting ligand through a chelator and linker. The linker connects the targeting ligand to the chelator and influences the radioligand's kinetics, while the chelator acts as a molecular bridge, forming a strong, tight bond between the isotope and the ligand. The ligand acts as the targeting vector, guiding the RLT to specific cancer cells, where the radioisotope delivers its therapeutic effect. The radiolabeling process ensures that the RLT is chemically stable and able to effectively deliver its therapeutic dose to the intended target.19
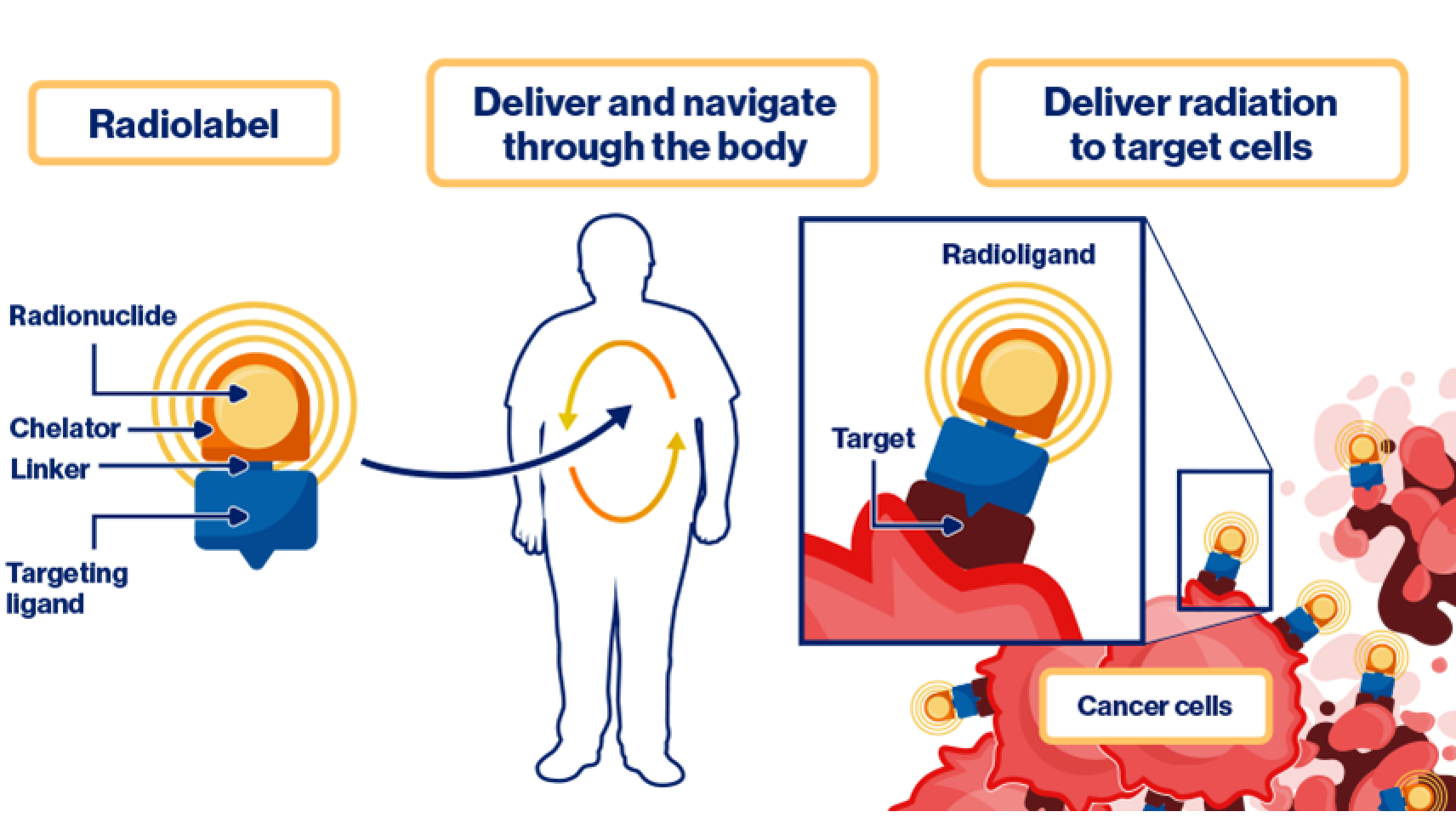
QA and QC of the RLT
Quality assurance (QA) and quality control (QC) are critical steps in the radiolabeling process to ensure the radioligand meets the required standards for safety, efficacy, and regulatory compliance.20 Due to short physical half-lifes, QC must be performed quickly and efficiently before use, verifying that all products meet strict predefined criteria for safety, accuracy, and efficacy.6