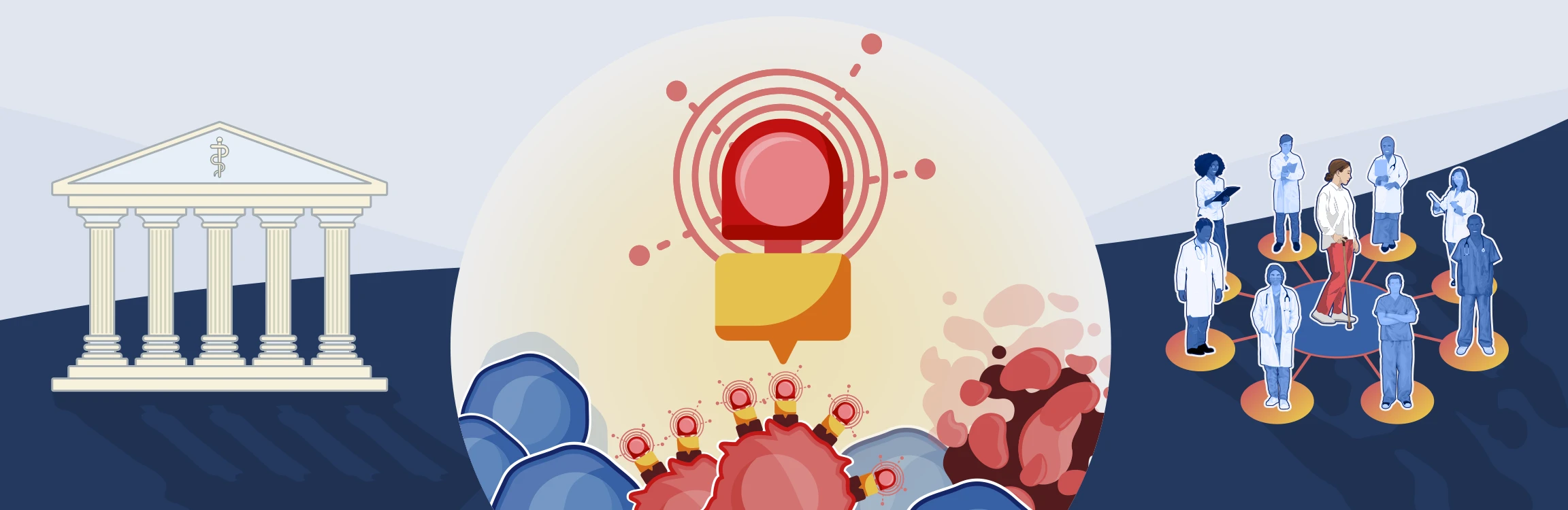
Foundations of theranostics
Radioligand therapy (RLT) and radioligand imaging (RLI) offer a novel, theranostic approach to targeting and treating certain types of cancer.1 This section of the Novartis RLT Institute is designed to help health care professionals (HCPs) understand the basic scientific foundations of RLT. By focusing on the science, we aim to demystify radiation and safety concerns and build confidence in offering patients this approach to precision medicine in cancer.
Radiation science fundamentals
Theranostic applications of radiation
Theranostics is the pairing of a diagnostic and a therapeutic radioligand to target the same biomarker for both imaging and treatment.2
Radioligand imaging (RLI): SPECT and PET
In nuclear medicine, single photon emission computed tomography (SPECT) and positron emission tomography (PET) are functional imaging methods used to visualize the distribution of radioligands within the body.2 To improve image accuracy, hybrid machines combine these functional imaging techniques with computed tomography (CT) imaging for detailed anatomical information. In SPECT/CT and PET/CT, the CT component is crucial for attenuation correction, compensating for tissue absorption that can distort functional images.3
SPECT
SPECT is a type of nuclear medicine imaging technique that provides 3-dimensional images by detecting gamma rays emitted from radioligands injected into the body.2 The gamma rays are detected by a rotating gamma camera, and the resulting data are used to generate cross-sectional images of the distribution of the radiolabeled compound.2,4 SPECT detects gamma-emitting isotopes such as 99mTc, 177Lu, 67Cu, or 131I.5,6
PET
PET uses positron-emitting isotopes like 18F, 68Ga, and 64Cu.7 These isotopes emit positrons (β+), which interact with electrons in the body, resulting in the production of 2 identical annihilation photons (511 keV each) directing in 180 degrees.4,8 PET scanners detect these photons and use them to create high-resolution quantitative images of metabolic and molecular processes within the body.3 PET generally provides higher resolution and more accurate images than SPECT due to the more precise detection of annihilation photons.5,9
For SPECT, we observe a range of photon energies emitted by different radionuclides. For example, for 177Lu, the significant photon energies are 113 keV (with 6% emission intensity) and 208 keV (with 10% intensity).10 Similarly, 67Cu emits photons at 93 keV (16%) and 185 keV (49%).11 These energy peaks are essential for optimizing imaging and detection systems, as they directly influence the sensitivity and resolution of the imaging system in nuclear medicine applications.10 In contrast, PET imaging relies only on the 511 keV photon energy from the 2 annihilation photons.7
By combining PET or SPECT imaging with targeted RLT, theranostics allows for precise tumor targeting.2 Both SPECT and PET imaging rely on radioligands to generate 3-dimensional images, but they differ in the type of radiation detected and how the images are produced:
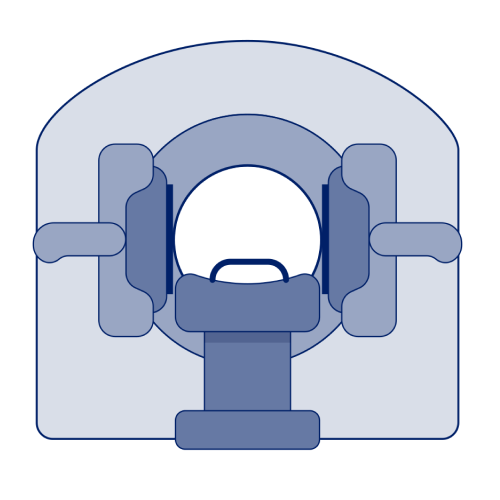
SPECT/CT
- SPECT/CT imaging instruments provide 3-dimensional (tomographic) images of the distribution of radioactive tracer molecules that have been introduced into the patient’s body4
- The instruments use gamma camera detectors to detect gamma rays emitted by the tracers injected into the patient to create detailed images4
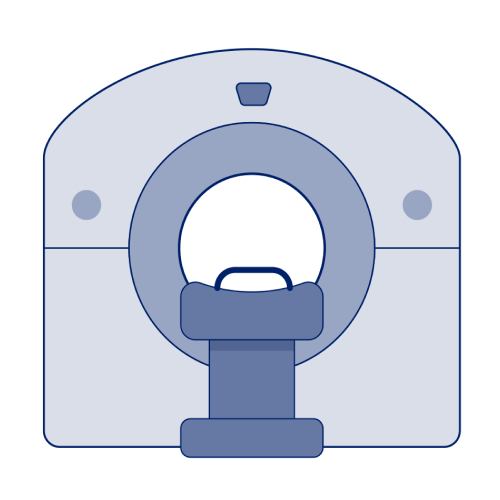
PET/CT
- PET/CT scans use RLI agents to create 3-dimensional images but rely on a different mechanism of action than SPECT scans4
- Unlike SPECT, which measures gamma rays, PET tracers, once injected, decay by emitting small particles called positrons4
RLT: A breakthrough in precision medicine
RLT represents an advancement in precision medicine, offering a targeted approach to treating cancer. By combining the specificity of biologic molecules with the potency of radionuclides, RLT delivers alpha and beta particles that interact with tissues via direct ionization along their path. This leads to DNA strand breaks—either single stranded or double stranded—that, if left unrepaired, can induce mutations or cell death. By harnessing ionizing radiation to induce lethal cellular damage, RLTs have become a powerful tool in modern oncology.12,13
Biologic effects and mechanism of action
DNA damage and cell death
Ionizing radiation directly damages DNA through chemical modification of DNA bases, single-strand breaks, and double-strand breaks, all of which can impair the cell's ability to survive.12
If repair mechanisms fail, the damaged cell undergoes cell death via apoptosis, necrosis, or other pathways such as mitotic catastrophe, or senescence.12,14
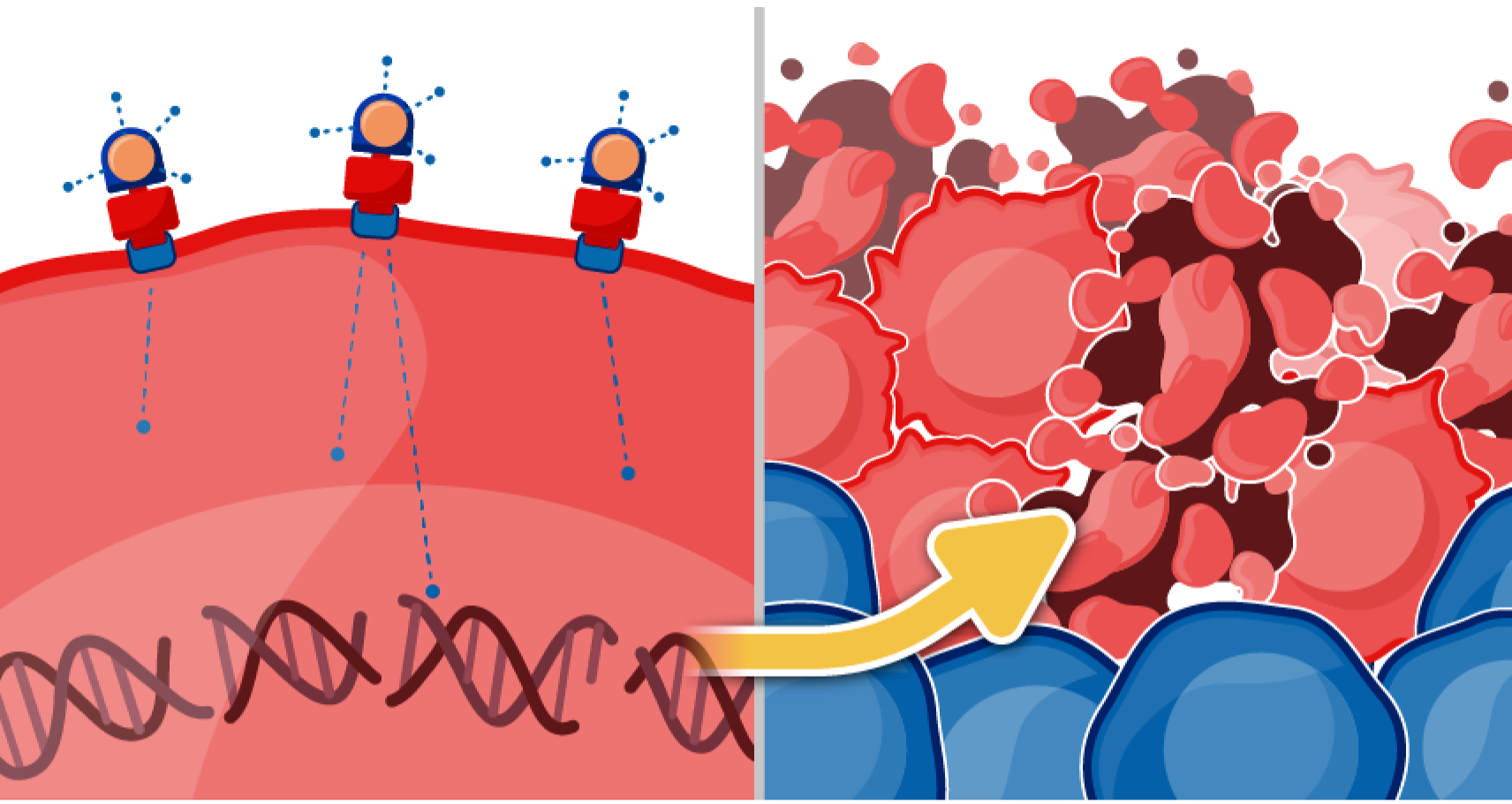
Cross-fire effect
Cross-fire irradiation is secondary damage caused when ionizing radiation emitted from target cells extends to nearby nontarget cells. For example, beta particles have a moderate range in tissue, enabling them to reach surrounding tumor cells.12,15
The cross-fire effect eliminates the need to target every cancer cell within a tumor, as radiation can effectively destroy neighboring cells within its range. However, the cross-fire effect can also induce unwanted tissue damage in nearby normal cells.12,15
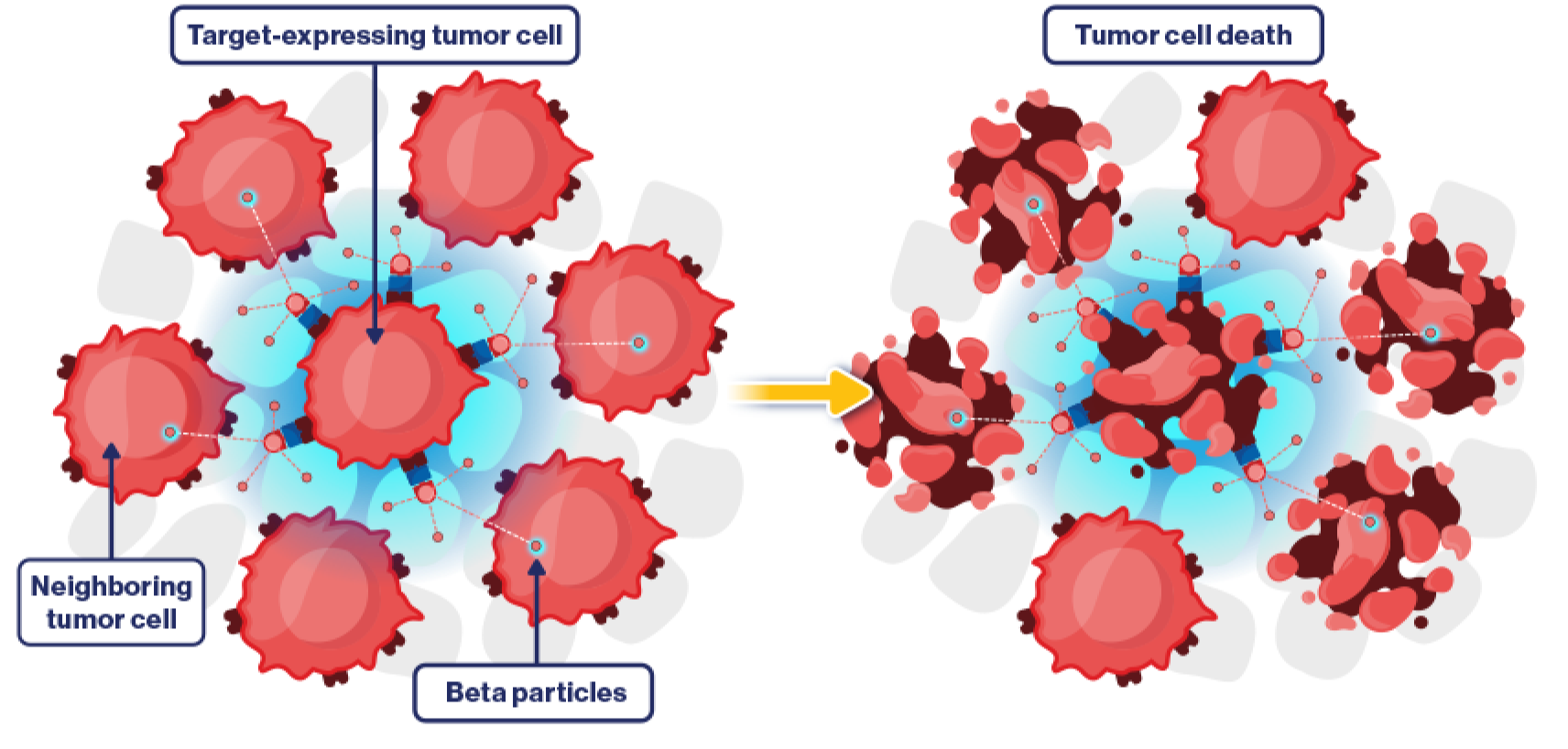